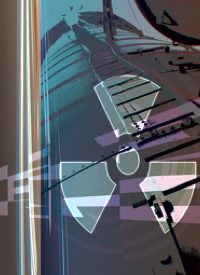
Editor’s Note: This article is the third in a continuing series published by The New American. For background material, please reference Dispelling Irrational Fear of Radiation and Understanding Radiation Risks & Benefits.
You may remember from high school chemistry class that atoms are made of protons, neutrons, and electrons. The graphic below depicts positively-charged protons and neutral neutrons (red), with negatively-charged electrons orbiting the nucleus.
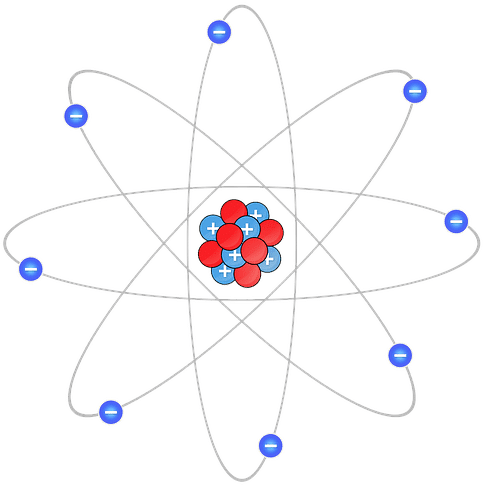
The number of protons defines the element and determines how elements are listed on the periodic table. Hydrogen is first because it has one proton. Helium takes second place with two protons. The image above depicts carbon since its atomic number is six. As for the rest, here’s a fun refresher from AsapScience.
Neutrons are different; the same element can have different numbers of neutrons. For example, hydrogen may have zero, one, or two neutrons. These different forms of hydrogen are called isotopes.
All chemical elements have multiple isotopes, some of which are unstable, or radioactive. In the latter case, they are called radioisotopes.
The Nuclear Family
Radioactive decay is the process by which an unstable atomic nucleus becomes more stable by emitting energy in the form of particles or ionizing electromagnetic waves.
The nucleus undergoing radioactive decay is called the parent nucleus and the nucleus resulting from radioactive decay is called the daughter nucleus.
The previous article in this series, Dispelling Irrational Fear of Radiation, identified alpha particles, beta particles, and gamma-rays as the types of ionizing radiation that originate from radioactive decay.
An alpha particle is a helium nucleus (two protons and two neutrons). Alpha particles are generally emitted when very heavy radioisotopes like uranium-235 (U-235) and uranium-238 (U-238) decay.
A beta particle is an electron. Nuclear reactor fuel fission products generally decay by beta particle emission.
Gamma-rays are electromagnetic waves that have no mass. Gamma-ray radiation accompanies alpha and beta decays when the resulting daughter nucleus is in an elevated energy state. Since a nucleus wants to be in its ground energy state (lowest energy), an “excited” nucleus will emit one or more gamma-rays to achieve this ground state.
Radioactivity
Just because a substance is undergoing radioactive decay does not necessarily mean it is dangerous. One of the determining factors is type of radiation (e.g., alpha, beta, or gamma), and another is timing.
Although it is impossible to predict the precise time at which the nucleus of a radioactive atom will decay, the average behavior of a very large number of radioactive atoms can be experimentally determined. The average time dependence for the decay of a specific kind of radioactive atom is called the decay constant, and is symbolized with the lowercase Greek letter lambda (λ). The decay constant is the probability per unit of time that a decay will occur.
A unit called activity, which is the product of the decay constant and the number of radioactive atoms present (λN), is used to characterize the radioactivity of a material. The larger the decay constant or the more radioactive material you have, the higher the activity.
You can also use the decay constant to determine half-life of radioactive atoms, which refers to the rate at which radioactive atoms decay. (The rate is exponential, and the formula used to calculate it is T½ = 0.693/λ.) Half-life is the average time required for the atoms to decrease to one-half of the initial number present.
After one half-life, only 50% of the initial atoms remain, after two half-lives only 25% of the initial atoms remain, etc. After ten half-lives only about 0.1% of the initial atoms remain. This behavior gives rise to the characteristic half-life curve as shown in the graphic below.
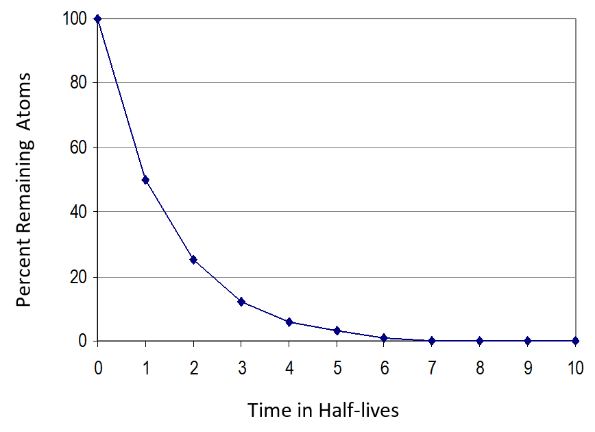
This graph illustrates that more short-lived atoms undergo radioactive decay than long-lived atoms in real time, resulting in higher activities. In other words, the higher the radioactivity, the shorter the half-life. Very long-lived isotopes like U-235 and U-238 have relatively low activities because they decay so slowly.
On the other hand, relatively short-lived radioisotopes (with half-lives on the order of a day or less) decay away fairly quickly. This becomes more apparent when the percent of remaining atoms is plotted in real time, rather than in half-lives, for radioisotopes with half-lives of 10 minutes, 50 minutes, 100 minutes, and 500 minutes, as shown in the following graphic:
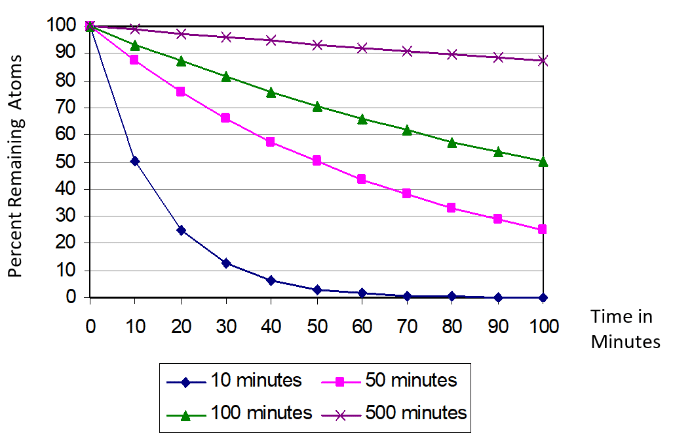
Exposure
Whereas activity (λN) characterizes the rate of radioactive decay for a radiation source (i.e., disintegrations per unit of time), dose rate characterizes the rate at which matter is exposed to radiation from a radiation source.
Dose rate for a specific radiation type (i.e., alpha, beta, or gamma-ray) depends directly on the radiation source activity, the radiation energy, and the radiation’s quality factor. (See Dispelling Irrational Fear of Radiation for a brief discussion of tissue weighting factor as a more appropriate measure of actual biological effect than radiation quality factor).
Dose rate also depends inversely on the distance of the exposed matter from the source. Therefore, the larger the distance from a radiation source, the smaller the dose rate. Dose rates are generally expressed in millirem per hour or milli-Sieverts per hour. (Click here for an explanation of rem and Sievert units of measurement.)
The dose absorbed by biological tissue then depends on the dose rate and the tissue exposure time.
Other Articles in This Series
Stay tuned for our next installment, when we will delve deeper into the topic of determining radiation dose, which will help you figure out what’s harmful and what’s helpful in the nuclear world, regardless of media bias.
Click the following for the previous articles in this series: Dispelling Irrational Fear of Radiation and Understanding Radiation Risks & Benefits
Jeffrey Mahn is a retired nuclear engineer, and currently volunteers as a docent and educator at the National Museum of Nuclear Science and History in Albuquerque, New Mexico.